Intro
Solar energy is hot. In times of increasing energy consumption, solar cells are considered one of the key technologies towards a sustainable energy supply. Especially now with more and more countries reaching grid parity, solar energy becomes a real alternative for conventional electricity production. Despite the rising enthusiasm, solar cell conversion efficiencies are still fairly low. The challenge remains to increase efficiency while keeping the production costs low. Recent work of María Recamán Payo, researcher at the silicon photovoltaics (PV) group in imec, tackles the issue by applying a process technology called ‘selective epitaxy’ on bifacial solar cells. By optimizing the doping profile on the emitter side, she has managed to reduce recombination, a major cause for efficiency loss. Moreover, because selective epitaxy is a simple, one-step process, device production costs are bound to be lower.
A question of efficiency
Although the sun is the most abundant source of energy on the planet, solar cells only convert a fraction of that enormous potential into electricity. In fact, most PV solar cells have a theoretical efficiency ceiling, limiting the amount of energy they can extract from the sun. But with current technologies, we are still far from hitting that ceiling. To put things into perspective: even though the upper-limit conversion efficiency for silicon devices is calculated to be around 30%, solar cells in commercial solar panels typically only reach an efficiency of around 18%. Most PV research is therefore focused on finding solutions to close this gap, while at the same time keeping production costs low. “The field of PV has already made tremendous progress, for example by improving junction profiles and passivation layers."
"However, to boost the efficiency of solar cells towards the theoretical limit, we need to address their recombination losses in the metallized region of the emitter; the region that harbors the contacts. That is the Achilles heel of current solar cells,” says Recamán.
When a ray of sunlight hits the photovoltaic material, it enters with sufficient energy to dislodge an electron from a silicon atom, increase its energy and leave behind a ‘hole’. The electron then travels to the corresponding contact region where it is harvested as electricity. That is, if no recombination events occur. When the electron that is on its way to the contact region encounters a hole, it will recombine and lose its energy. Recombination effectively cancels out the charges’ contribution to the electrical current, and is thereby one of the fundamental factors that limits efficiency. Different recombination mechanisms can occur in parallel. The so-called Auger recombination involves three charge carriers. In this case, the excess energy given off by an electron recombining with a hole is passed on to a second electron, instead of being emitted as heat or a photon. For silicon solar cells, it is the dominant process in the highly-doped regions, the regions where charge carrier concentrations are the highest, such as the contact area.
Selective epitaxy to combat recombination
With a procedure called selective epitaxy, Recamán found a way to selectively adapt the doping profile in the metallized region to minimize Auger recombination and ultimately increase cell efficiency. “Epitaxy is a relatively new technique in solar cell fabrication; a technique in which you grow a micron-thin layer on a silicon substrate. Specifically for homo-epitaxy, a silicon source material reacts with p- or n-type dopant sources in the presence of a silicon substrate surface. The resulting layer grown on the surface displays the same characteristics as the substrate, defects included.
Epitaxy is much like applying a skin on a wafer template.
This is very different from diffusion, the gold-standard technique for doping in industry. In that case, dopant atoms are driven into the substrate during high-temperature processing.”
Epitaxy has many advantages that make it a promising technique for fabrication of highly efficient and cost-effective solar cells. First of all, epitaxy is a more versatile and flexible process to create specific profiles depending on the application. Because the doped layer is applied following a box profile, thickness and dopant level can be optimized independently, whereas doping by diffusion with a typical Gaussian profile requires simultaneous adjustments of both parameters. Secondly, after epitaxy, the p/n-junction is ready to be used. Diffusion, however, induces the formation of a ‘glass layer’ on the doped surface: a byproduct that needs to be etched away. Other techniques such as ion implantation also require extra high-temperature annealing steps to activate the dopants. The fact that the final doping profile can be achieved in one step using epitaxy is a big plus for overall production costs. To accomplish the same results using diffusion would require a more complex strategy, involving more steps and thus higher costs.
A precision process for boosting solar cell efficiency
According to Recamán there is one advantage that stands out: “The most important benefit of epitaxy compared to traditional diffusion is its selectivity. During solar cell fabrication, a dielectric is patterned on the wafer, leaving areas of silicon exposed to accommodate the contacts later on. The technique of selective epitaxy will only target these open sites, leaving the surroundings undisturbed. After epitaxy, the dielectric can then still perform its function of passivation or antireflection layer.”
It is exactly this selectivity that can make a difference in the metallized region where high doping levels are needed to achieve a low contact resistance, contrary to the passivated region where too high doping levels might enhance Auger recombination. “Selective epitaxy can decouple these two issues by creating a specific doping profile with independently optimized doping level and thickness in each of the two emitter regions.
That way, a thick, highly doped layer at the contact region can strengthen the contact, and a lowly doped layer at the rest of the surface helps to reduce Auger recombination. All in a simple step; this would be impossible using diffusion.”
Recamán has already applied epitaxy to test structures without contacts. “The next step would be to apply the metallization and make the final cell. Then we can measure the conversion efficiency in that device and show that epitaxy can outperform standard techniques.” And expectations are high: for epitaxy on silicon monofacial devices, an efficiency of 22.5% was already established. Now, with bifacial cells that allow light to be captured from both sides, silicon epitaxy at the contact regions has significantly reduced recombination. For structures before metallization that have a thick, highly-doped epitaxial layer at the contact region, the dark saturation current density (a measure for the total recombination) totaled at 55 fA/cm², while the best diffusion-based cells still reach 70 fA/cm². A lower recombination loss also has an impact on the open circuit voltage (VOC) that determines how much electricity can be maximally extracted from the system. “Bringing down the recombination losses in the metallization region is very important for obtaining a high open circuit voltage. The best VOC achieved to date for diffused bifacial solar cells is 688 mV."
"With the current structures, we are expecting a much better VOC in the range of 695 mV. We even hope to reach the barrier of 700 mV,” concludes Recamán.
The future shines bright
The versatility of selective epitaxy to fine-tune the doping profile in the emitter regions paves the way for an additional increase in solar cell efficiency. On top of that, because of the simplicity of the process, the energy production would also be more cost-effective. The technology, therefore, holds an enormous potential for being used in solar panels on rooftops or in specialty installations, both the monofacial and bifacial varieties.
With solar energy becoming more cost-effective, it will take up an ever-larger share of the earth’s energy portfolio. Research into renewable energy sources has therefore an important place on imec’s R&D agenda. Examples are initiatives such as Flanders’ EnergyVille (Genk). EnergyVille unites the unique expertise from KU Leuven, VITO, UHasselt and imec into one big laboratory where researchers work on the sustainable energy systems of the future. The solar cells that are fabricated and manufactured in imec will be integrated in modules and systems in EnergyVille, where also further testing takes place to ensure that the final devices are ready for a sunny future.
Want to know more?
- Find out more about María Recamán Payo’s work in her recent publication (mail to imecmagazine@imec.be for a copy)
- Learn more about EnergyVille on their website
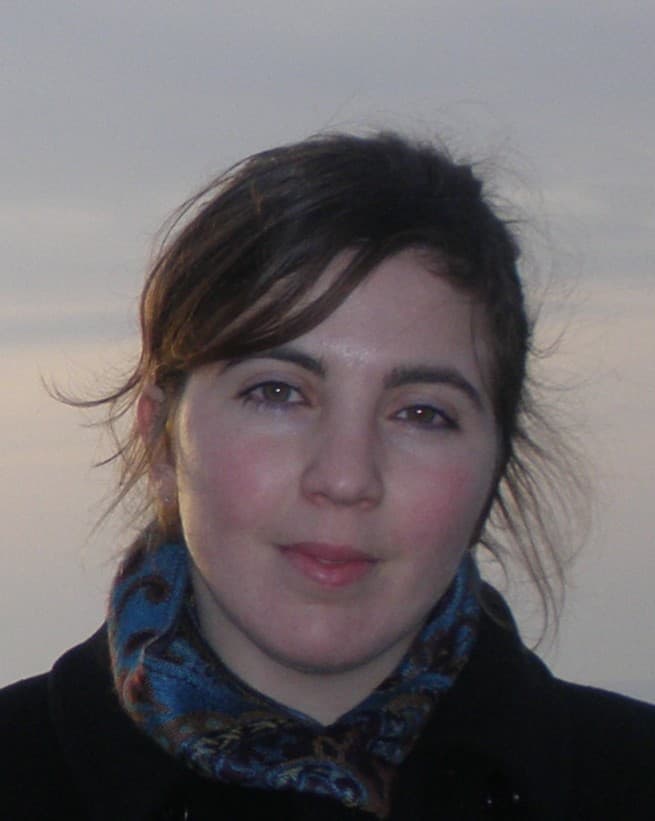
María Recamán Payo received the degree in Chemical Engineering from the Universidad de Santiago de Compostela in 2002, followed by the PhD degree from the Universidad Complutense of Madrid in 2008. In that same year, she joined imec where she is currently working as a senior scientist in silicon photovoltaics. Her domain of expertise is the application of silicon epitaxy and polysilicon growth by thermal CVD (Chemical Vapor Deposition) for junction formation and passivated contacts in bulk crystalline silicon solar cells.
Published on:
26 October 2017