2D materials: an emerging material class...
Materials characterization/metrology are key enablers in the semiconductor industry as they not only support fundamental R&D but also provide a means to ensure that high volume manufacturing (HVM) is proceeding as spec’d. And with Moore’s law continuing its relentless path forward (3nm logic process flows are expected to go into production mid 2022/early 2023 with 2nm due shortly thereafter), the question is: how small can we go?
One of the barriers to further downscaling concerns electrical leakage in the form of short channel effects. To contend with this, novel new solid-state materials and architectures are continuously being explored.
And with this, 2D materials, which includes graphene, transition metal dichalcogenides (TMDs) and topological insulators (TIs), are now appearing on various technology roadmaps. That means the needed R&D needs to happen now.
2D materials are of interest since they display attractive carrier mobilities along with reduced leakage issues. Graphene (a 2D carbon allotrope) for example, may potentially be used in future logic devices (carbon-nano-ribbons open a bandgap), memory devices, opto-electronic devices and/or interconnects. As for TMDs, examples such as tungsten disulfide (WS2) and molybdenum disulfide (MoS2) are being looked at as potential replacements of silicon (Si) in the conduction channel of logic devices as well as opto-electronic devices. And like graphene, TMDs can be arranged as multi or mono-layer structures.
And lastly, much is anticipated from 3D topological insulators (TIs), a material class that was first theorized in 2005 and for which the 2016 Nobel Prize in Physics was awarded. 3D-TIs behave like an insulator in their interior, but their surface contains spin locked conductive states, i.e., all electrons travelling in a given direction will have the same spin. Furthermore, these will be protected from defect scattering. Along with potential applications in logic and memory devices realized, TIs may also provide a means to de-coherence free quantum computing with the bismuth selenide (Bi2Se3) form taking center stage, at least for the moment.
Pictorial images of a) the crystal structure of Bi2Se3 and b) a Dirac point
The unique properties of TIs stem from their topological properties or more precisely, the existence of “Dirac points” in their electronic band structures. These “Dirac points” are formed at energy levels near the Fermi edge and take the shape of upper (conduction) and lower (valence) halves of a conical surface, hence are referred to as “Dirac cones”.
... with new characterization needs
The performance of any electronic device reliant on atomic scale engineering is governed by such things as chemical composition inclusive of dopant levels within the respective films as well as interface quality. And the continued downscaling is pushing the limits of what is possible with existing characterization capabilities, whether used in the lab or the fab.
Today, lab-based solid-state characterization is mainly centered around the imaging techniques of scanning electron microscopy (SEM), transmission electron microscopy (TEM) and those within the scanning probe microscopy (SPM) family inclusive of atomic force microscopy (AFM). There is also a plethora of spectroscopic and spectrometric techniques used such as: infra-red (IR), Raman, x-ray photoelectron spectroscopy (XPS) and variants thereof, Rutherford back scattering (RBS), secondary ion mass spectroscopy (SIMS), atom probe tomography (APT), and the list goes on.
2D materials, however, bring new analytical requirements and challenges, with examples including being able to measure electron spin, magnetism, and the electron band structure.
Time resolved (tr) techniques will also be needed to understand the dynamics of such things as electron spin flipping as this plays a central role in spintronics based devices.
Leveraging HHG sources and the AttoLab
With this, and interference lithography in mind, imec started in 2019 a joint development project with KMLabs and SPECS to create what is referred to as the “AttoLab”.
In 2020, two table-top high-harmonic generation (HHG) extreme ultraviolet (EUV) sources fed by a high powered femto-second titanium-sapphire laser were made operational. These HHG sources provide bright coherent pulsed photon beams. One of them feeds a table-top imaging/spectroscopy beamline with 25-120 eV (50-10 nm) photons, while the other feeds a table-top EUV interference lithography (EUV-IL) beamline with 92 eV (13.5 nm) photons. An IR spectroscopy end-station was also setup on the imaging/spectroscopy beamline. And lastly, low energy electron diffraction (LEED), angle resolved photo emission spectroscopy (ARPES) and photo electron emission microscopy (PEEM) instrumentation was installed.
Moving forward, the imaging/spectroscopy beamline will be extended to the ARPES/PEEM chamber. This beamline will also support coherence diffractive imaging (CDI) and magneto optical Kerr effect (MOKE). And lastly, the introduction of pump-probe photon beams will facilitate tr studies. Recognition of the value of tr-IR and tr-ARPES is highlighted by the fact that Kevin Dorney and Dhirendra Pratap Singh were both awarded Marie Skłodowska-Curie Actions grants in 2021 for their AttoSPIE and AttoPES projects.
In the longer term, additional capabilities ranging from EUV magneto circular dichroism (MCD), MCD-PEEM, MCD-CDI to various forms of scatterometry and reflectometry are foreseen. The eventual vision is to push the limits of HHG sources such that fully coherent ultra-fast pulsed photon beams up to 1.2 keV (1 nm) are realized. This has the potential to drive CDI to nm scale spatial resolution while also enabling the implementation of near edge x-ray absorption fine structure (NEXAFS), i.e., techniques typically confined to synchrotron sites. This highly accessible table-top facility will thus serve to enhance time-to-data from months to days.
What will these capabilities bring to imec?
IR, ARPES and MOKE (in its traditional form), are spectroscopies that are sensitive to valence shell electrons, with IR providing information on molecular structure, ARPES mapping the valence band structure (ARPES was the 1st technique to empirically map Dirac cones in 2007), and MOKE for measuring magnetization. The implementation of tr capabilities, via pump-probe methods, takes these spectroscopies to the next level as this allows for the kinetics to be followed, i.e., the pump-photon induces a change for which the probe photon following a short time later will measure. As an example, pumping electrons into unoccupied levels above the Fermi edge will allow for Floquet-Bloch states among other things, to be examined (this is the topic of the AttoPES tr-ARPES project). Comparison of tr-IR and tr-ARPES spectra with those obtained via ab-initio calculations within imec will thus provide a deeper level of understanding of processes active to femtosecond regimes and beyond.
Increasing the energy of the incoming photons into the EUV region will allow for the examination of shallow core level electrons via the implementation of EUV-MOKE and EUV-MCD. And since the energies of core electrons scale more closely with the number of protons in the nucleus, this will provide information on the element responsible for magnetization. Indeed, with photon energies up to 120 eV one will be able to probe 1st row transition metals inclusive of Fe, Co, and Ni. To probe deeper core levels will require even higher energies.
And with the long-term vision extending to 1.2 keV, this would be realized. 1.2 keV will also facilitate the implementation of NEXAFS, providing detailed information on elemental speciation, i.e., the chemical compound formed.
As for LEED, PEEM and CDI, the former is a crystallographic technique, while the latter two are imaging techniques. PEEM maps the locations of photoemissions from a solid to a spatial resolution in the 20-100nm range (70nm in the AttoLab system), and thus provides ARPES with the ability to examine a specific region of interest. Likewise, LEED ensures the film of interest has been prepared in the correct manner prior to PEEM/ARPES experiments (all are within the same instrument). Lastly, CDI relies on the phase shift experienced by a coherent photon beam directed at a solid. This can provide SEM and AFM like images to a spatial resolution better than PEEM, but not yet in the realm of SEM and AFM (~1 nm for both). And since CDI resolution scales with wavelength, it is of interest to see what can be attained at 1.2 keV (1 nm), while also pushing scatterometry/reflectometry possibilities. And to examine magnetized regions to unprecedented spatial resolution (down to a few nm or less?) one can envisage the application of MCD-PEEM, or even MCD-CDI.
ARPES: a first characterization milestone
Recently, the AttoLab team hit one of its first milestones by observing, via ARPES, the Dirac cone in imec fabricated Bi2Se3 topological insulator films. Although Bi2Se3 has potential applications in logic and memory devices, this work was carried out in the context of developing a next generation of de-coherence free qubits. These qubits, called Majorana qubits, are the subject of the NOTICE project for which imec’s Clement Merckling was awarded a 5-year ERC Consolidator Grant in 2019. The observation of the Bi2Se3 Dirac cone not only proves that the fabricated material behaves as a topological insulator, but will also enable the tailoring and refinement of Bi2Se3 processing. For this proof-of-concept experiment, the He discharge source within the ARPES instrument was operated to provide a continuous source of photons at 21.2 eV.
Observation of the Dirac cone using ARPES on an imec fabricated Bi2Se3 topological insulator. EF refers to the Fermi edge, CB the Conduction Band, DP the Dirac Point and VB the Valence Band.
Low-energy electron diffraction (LEED) experiment carried out in the ARPES preparation chamber.
Conclusion
Table-top high-harmonic generation sources can enable a variety of imaging and spectroscopic capabilities that will be key for the characterization and fundamental understanding of emerging materials, inclusive of 2D materials. When implemented in an industry relevant laboratory – such as the AttoLab – they will accelerate the development of next-generation logic, memory and quantum devices. With the first observation via ARPES of a Dirac cone within imec fabricated Bi2Se3 films, the AttoLab is well on course to realize the R&D goals laid out during its inception.
Acknowledgements
This work is the result of the collaborative effort of the imec AttoLab team, including – from left to right – Esben Larsen, Paul van der Heide (Co-AttoLab Principal), Dhirendra Singh, John Petersen (Co-AttoLab Principal), Thomas Nuytten, Kevin Dorney, Fabian Holzmeier and Michiel van Setten.
the imec AttoLab team
Want to know more?
- AttoLab serves a second, equally important but different purpose: accelerating the development of high-numerical-aperture (high-NA, i.e. 0.55NA) extreme ultraviolet (EUV) lithography, by providing new insights into the way the 13.5nm photons interact with the photoresist. For more info, read imec’s press release ‘Imec demonstrates 20nm pitch line/space resist imaging with high-NA EUV interference lithography’.
- ‘Surface analysis in the semiconductor industry: present use and future possibilities’, P.A.W. van der Heide et al, Surf Interface Anal. 2020; 52: 786-791;
- ‘Imec introduces 2D materials in the logic device scaling roadmap’, imec reading room;
- ‘Prestigious European ERC consolidator grant awarded to imec’s Clement Merckling for developing fault-tolerant qubits’, imec press release;
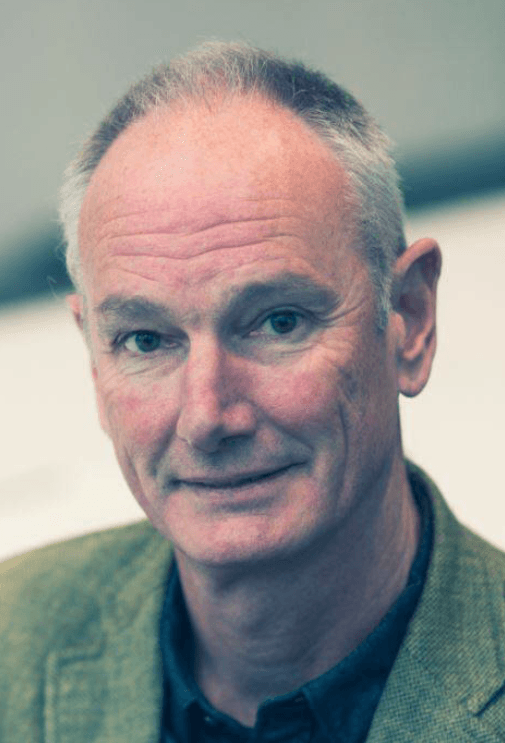
Paul van der Heide is the Director of the Materials and Component Analysis (MCA) department and is Co-AttoLab Principal at imec in Leuven, Belgium. The scope of MCA is a) to support the materials characterization needs of the imec R&D facilities, and b) to explore, develop and implement cutting-edge characterization capabilities required to support tomorrow’s micro-electronics industry. Prior to moving to imec, Paul held positions at GLOBALFOUNDRIES, Malta, NY, (where he headed the analytical labs end-to-end (chemical, physical, and electrical) support of CMOS manufacturing and R&D), Samsung, Austin, TX, (where he established and managed the surface analysis lab for supporting high volume CMOS manufacturing), and the University of Houston, (where he lectured graduate and undergraduate courses in physical chemistry and surface analysis, while also managing the MRSEC SIMS-XPS facility). Paul earned a PhD in Physical Chemistry from the University of Auckland, New Zealand, has authored over 100 publications in international peer reviewed scientific journals, and is sole author of two books published through Wiley.
Published on:
22 March 2021