A number of prototypes have been tried and tested, with growing success. But what is still lacking are the electronics that can interface between the patients’ nerves and the new arm so that they feel like it is their own: supersmall implantable electronics that pack enough intelligence and electrodes to allow a fine-grained contact between the prosthetics and the hundreds of available nerves. A new implantable chip designed at imec may prove to be that missing link, explains Dries Braeken, R&D manager at imec.
When someone loses an arm in a car or industrial accident, they are left with a stump. But their brains’ primary motor cortex still holds all the circuitry that used to drive that arm with its hand and fingers. That circuitry is the brain’s image of that arm. It creates what many patients experience as ghost itches and pain in a limb that is no longer there. But it also keeps sending signals to move the hand and fingers. When patients inadvertedly want to shake someone’s hand or catch a ball that is thrown at them, the motor cortex initiates burst of electricity. These are passed down through the spinal cord, into the nerves that still serve the stump, and from there into the remaining muscles, which can be seen twitching.
All that is needed to help these patients, it seems, is to make a sufficiently sophisticated artificial arm with electromotors and sensors and fuse it with the stump.
To do so, however, a major multidisciplinary effort is required. It involves e.g. developing superlight and strong mechanics, integrating electromotors that allow the same forces and flexibility as human muscles, developing a biocompatible interface with the stump, and translating between human-generated and artificial signals.
A first generation of such smart prosthetics was designed to pick up the electrical signals from the remaining muscles, using these to drive the electromotors in the artificial arm. But patients equipped with such an artificial arm often experience it as a dead weight, an object that not really belongs to them. And therefore, they’d often rather not use it.
There are a number of reasons why they wouldn’t.
For one, these prosthetics do not allow the superfine finger control with which people interact with their environment and which they have learned through decades of finetuning. Patients can pick up a pen but they cannot write.
But even more important is the missing feedback. The artificial limb is not able to signal to the brain if it is touching a soft surface, picking up a burning coal, or applying just enough pressure to hold that Starbucks coffee cup but not crush it.
To create a more natural prosthetics experience, DARPA (the research agency of the USA army) set up the HAPTIX program, shorthand for ‘Hand Proprioception and Touch Interfaces’. The goal of the program is to create a prosthetic hand that moves and provides sensations just like a natural hand. It wants to do so by interfacing directly with the nerves instead of the muscles, to connect the biological and artificial circuitry through a permanent, implanted link that sends and reads electrical signals in two directions.
The nerves that run through our body are bundled and shielded in nerve fascicles, resembling how e.g. control and communication wiring in a building is bundled in a cable tray. Our arms and hands are controlled by two such bundles, called the median and ulnar fascicle. One promising technique that is developed and tested by HAPTIX is to integrate electrodes in a collar that fits around the 5mm thick bundles. But the challenge is to read and stimulate from the exact right nerves in the bundle without contacting them, nerves moreover that move and slide inside the fascicle. So there is an upper limit to the precision that can be reached with this method.
A more precise approach might be to insert electronics into the fascicle to contact the individual nerves. It’s a method that carries more risk, needing precise microsurgery that places electrodes inside the fascicle but doesn’t damage the actual nerves. Four years ago, a first such electrode was implanted temporarily in a patient (https://actu.epfl.ch/news/amputee-feels-in-real-time-with-bionic-hand/). The test was successful but showed the need to create compact implantables that include much more intelligence and tightly-spaced contact points, i.e. electrodes that stimulate and read the individual nerves.
That is where the recently unveiled imec chip comes in.
Manufactured with the same silicon technology that has been fine-tuned to make today’s advanced computer chips, the new biochip is only 35um thick, thinner than an average hair. On its surface are 64 electrodes that allow stimulation and recording of nerves, with a possible extension to 128. Through a needle attached to the chip, the package can be precisely lodged inside a nerve bundle so that the electrodes come into close contact with individual nerves.
Imec’s experts made the chip in close collaboration with their colleagues from the University of Florida, a main contractor under the HAPTIX program. They selected imec because it offers two unique advantages over other R&D facilities. Unlike most R&D labs involved in chip technology, it also has the equipment and processes in place to do actual high-quality manufacturing. And unlike most other manufacturing sites, it has the flexibility to set up dedicated manufacturing processes for such innovative designs.
What was actually the biggest challenge was not manufacturing the chip itself but rather integrating it with the package. In recent years, imec has developed a series of comparable biochips, think of neuroprobes with hundreds of electrodes along a long flexible shaft. So its researchers knew how to design the prosthetics chip. But until now, they had never created a hermetically sealed, biocompatible and flexible package to serve as a long-term human implant.
To do so, the engineers sandwiched nanolayers with superior barrier properties with very thin flexible polymer layers. The final result is an ultrathin flexible electronic device with a thickness comparable to that of a human hair and suitable for minimally invasive implantation.
The first phase of the project has been successfully concluded, and will now be followed by a testing phase in which the prototype will be manufactured in larger volumes. The new prosthetics chip will then be tested at the University of Florida and possibly also at other labs, mainly to see how it behaves in a biological environment – if it remains sealed and functioning for an extended period. In the meantime, researchers can start looking how to convert all the signals from the nerves into useful signals for the prosthetics. And in the other direction, they should figure out which useful signals from the prosthetics’ sensors they can inject in the nerve system and where precisely these signals should go.
These studies are a first step towards a sensory-enhanced prosthetic. Although it will take another few years to develop a commercially available arm, results like those of the University of Florida and imec show that this type of prosthetic can be made, that patients will eventually get prosthetics that feel more embodied, and that even the full bionic prosthetics of science fiction movies may one day become a reality.
Want to know more?
This work was sponsored by the Defense Advanced Research Projects Agency’s (DARPA) Biological Technologies Office under the auspices of Dr. Doug Weber through the Space and Naval Warfare Systems Center, Pacific Grant/Contract No. N66001-15-C-4018 to the University of Florida.
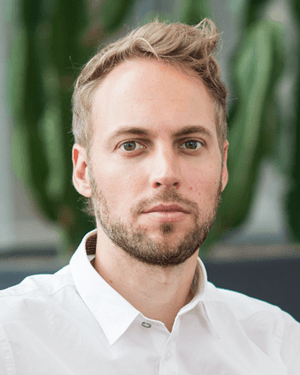
Dries Braeken obtained his Masters degree in biomedical sciences and his PhD in medical sciences from the KU Leuven, Belgium, in 2004 and 2009 respectively. From 2009 to 2012 he worked as senior scientist in imec's bioelectronics group. In 2012 he went on to become R&D team leader in the life sciences technology department, and in 2017 he became R&D manager and group leader.
Published on:
22 August 2017