The promise of superconducting qubits
Quantum computers promise to dramatically affect selected application fields, including materials synthesis, pharmaceutical drug development, and cybersecurity – to name a few.
In the quantum circuit model of computation, a quantum logic gate (or simply quantum gate) is a basic operation on a small number of qubits, which is analogous to a classical logic gate for conventional digital circuits. Qubits are the building blocks of quantum circuits. Different quantum computing platforms with diverse types of qubits are being developed and worldwide efforts are ongoing to bring them from the lab to the world.
One of the promising technologies for quantum computing makes use of superconducting circuits. Anton Potočnik, senior researcher in quantum computing at imec: “The energy states of superconducting qubits are relatively easy to control, and, throughout the years, researchers have been able to couple an increasing number of qubits together. This enables an ever-higher level of entanglement – which is one of the pillars of quantum computing.
Research groups worldwide have demonstrated superconducting qubits with long coherence times and sufficiently high gate fidelities.
On top of that, research groups worldwide have demonstrated superconducting qubits with long coherence times (up to several 100 µs) and sufficiently high gate fidelities – two important benchmarks for quantum computation.” While coherence time gives us information on how long a qubit retains its quantum state (and hence, its information), gate fidelity quantifies the difference in operation between an ideal gate and the corresponding physical gate in quantum hardware.
Large-scale implementation hindered by variability issues
The encouraging results mentioned above have so far only been obtained at lab scale, using double-angle evaporation and lift-off techniques for making the most critical element: the Josephson junction. “The superconducting qubit is essentially a non-linear LC resonator circuit, containing a non-linear inductor (L) and a capacitor (C),” explains Anton Potočnik. “The Josephson junction takes the role of a non-linear, non-dissipating inductor, which allows us to manipulate qubit energy states to represent, for example, a superposition of I0> and I1>. To minimize any losses of energy or, in other words, maximize coherence time, the various interfaces contained in the structures that make up the junction and the capacitor must be as clean as possible. Even one atomic defect present at one of the interfaces can cause the qubit to lose energy. And that’s why double-angle evaporation and lift-off are the preferred fabrication techniques: they can provide these extremely clean interfaces.”
The existing techniques for fabricating superconducting qubits challenge a further upscaling towards larger numbers of qubits.
But these fabrication techniques have a serious downside: they challenge a further upscaling towards larger numbers of qubits. Large-scale implementation is hindered by the variability in Josephson energy of the evaporated junction. In addition, the fabrication technique limits the choice of the superconducting material, and hence, the potential for qubit improvement.
An alternative approach using CMOS-compatible fabrication techniques
Jeroen Verjauw, PhD researcher at imec: “Our team at imec has explored alternative ways of fabricating the superconducting circuits. Our focus was on creating so-called overlap Josephson junctions using only CMOS-compatible materials and techniques, as this enables leveraging the reliability and reproducibility offered by state-of-the-art CMOS processing steps to control variability and facilitate upscaling.”
Imec’s focus is on creating overlap Josephson junctions using only CMOS-compatible materials and techniques.
Overlap junctions have two electrodes (bottom (BE) and top (TE)) separated by a thin insulator layer. The electrodes are defined in two patterning cycles, with a vacuum break in between. The break introduces uncontrolled growth of native metal oxide, which must be removed during a so-called Ar-milling step. “This Ar-milling step is however known to be very critical and has previously been reported to introduce unwanted energy losses,” adds Jeroen Verjauw.
Cross-sectional illustration of the overlap junction. The overlap between the bottom electrode (BE) and the top electrode (TE) defines the Josephson junction (and a parasitic stray junction). Sidewall residues can be present due to subtractive etching steps. The green layer represents the Ar-milling induced damaged amorphous Si layer.
Coherence times up to 100 µs, gate fidelity of 99.94%
Tsvetan Ivanov, researcher at imec: “We have demonstrated in our lab superconducting qubits with coherence times exceeding 100 µs and an average single-qubit gate fidelity of 99.94%. These results are comparable with state-of-the-art devices, but, for the first time, have been obtained using CMOS-compatible fabrication techniques – such as state-of-the-art sputtering deposition and subtractive etch. These breakthrough results could be achieved by improving the known process for making the overlap junctions. The improvements include process optimization to reduce the number of process steps and interfaces (and hence, the risk for energy losses), an improved Ar-milling step, and the exclusive use of aluminium (Al) for making the electrodes.”
(Left) Qubit energy relaxation measurement and (right) average gate fidelity and average error per gate.
The next steps: 300mm fabrication, reducing the losses and addressing reproducibility
Our experiments described in NPJ Quantum Information have so far only been achieved in a lab environment, on substrate coupons. Tsvetan Ivanov: “Yet, the presented fabrication method heralds an important milestone towards a manufacturable 300mm CMOS process for high-quality superconducting qubits. Soon, we will transfer the fabrication of these superconducting circuits into imec’s 300mm fab. We are eager to verify whether the high coherence times can be reproduced on larger wafer substrates.”
Soon, we will transfer the fabrication of our superconducting circuits into imec’s 300mm fab.
Jeroen Verjauw: “In addition, we designed our test vehicles such that we can study where the energy losses come from. First results have indicated that the losses mainly occur at the outer surface of the structure, and not, at the critical junction level. This is encouraging, as it leaves room for optimization by applying more dedicated surface treatment steps. And, finally, our fabrication method provides a path towards fabricating reproducible qubits over a large wafer area, with low variation in for example qubit frequency.”
Yet, there are other obstacles on the road towards practical superconducting-based quantum computers. Anton Potočnik concludes: “Superconducting qubits are still relatively large (mm-sized) compared to for example semiconducting spin qubits (nm-sized). We investigate how we can further shrink the devices. Many efforts are also ongoing on the algorithmic side. The qubits that we make today are not ideal, so there is a huge effort from the theoretical side to develop algorithms that are more resilient to losses and errors, and to develop quantum error correction protocols. On top of that, our community will need scalable, very well calibrated instrumentation to interface with the growing number of superconducting qubits, to control them and readout meaningful results.”
Conclusion and outlook
Kristiaan De Greve, program director quantum computing at imec, sees this work by Anton, Tsvetan, Jeroen and their coworkers as a crucial milestone towards being able to overcome fundamental barriers to upscaling of superconducting qubits by virtue of the control and accuracy benefits of industry-standard processing methods: “As many thousands to millions of physical qubits will likely be required for the quantum processors of the future, overcoming limitations due to variability and low yield will be crucial. Imec therefore invests significantly in understanding and benchmarking these limitations and introducing novel solutions that leverage our experience in advanced process control.”
Overcoming the limitations due to variability and low yield will be crucial.
Danny Wan, program manager quantum computing at imec, adds: “Within imec’s program on quantum computing, our scientists have set themselves the challenge to bring quantum computing (both semiconducting and superconducting based) from the lab to the world. Results as described in NPJ Quantum Information are extremely encouraging and confirm that we are on the right track in pursuing our mission.”
This article was originally published in EETimes.
Want to know more?
More details can be found in the article ‘Path towards manufacturable superconducting qubits with relaxation times exceeding 0.1ms”, by Jeroen Verjauw et al., NPJ Quantum Information, August 2022.
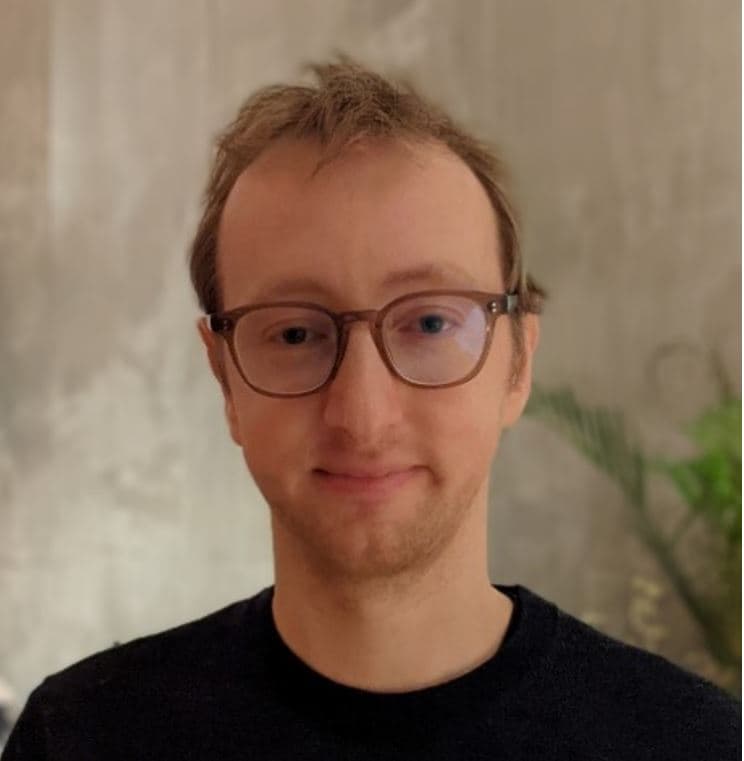
After obtaining his bachelor's in electrical engineering at KU Leuven in 2012, Jeroen Verjauw entered the Erasmus Mundus Master program in nanoscience and nanotechnology. During the second year, he studied at Chalmers tekniska högskola, Sweden, and worked in the group of Per Delsing to investigate the transmission properties of a two-qubit system. After obtaining his degree, he continued his studies at KU Leuven to further specialize in physics. In 2017, he obtained his Master of physics after investigating the superconducting properties of Sn nanowire arrays under the supervision of Kristiaan Temst, Margriet J. Van Bael, and André Vantomme. Currently, Jeroen is employed as a PhD researcher in the quantum computing team at imec and investigates qubit and resonator losses that arise from different fabrication and processing techniques.
Tsvetan Ivanov is a researcher in imec where he is developing lab-based solutions for quantum computing devices, exploring the path towards large scale integration.He defended his PhD on the subject of surface and interface properties of III-V nanostructures in Sofia University, Bulgaria in 2009, where he was an assistant professor till 2012. Tsvetan then joined imec’s III-V and Ge gate-stack team as a postdoc in the group of Dennis Lin. His research continued in the III-V vertical nanowire MOSFET project, exploring the limits of this technology. Since 2017 he is active in imec’s quantum computing team, -working on both spin and superconducting qubit devices.
Anton Potočnik is a senior researcher at imec where he is exploring quantum computing based on superconducting circuit technology. His main topics of interest are investigating sources of decoherence in qubits, interfacing qubits with cryo-CMOS electronics and exploring novel techniques for scaling up superconducting quantum devices. Before joining imec, Anton was a postdoc at ETH Zurich in prof. Wallraff’s group, where he worked on analog quantum simulations. One of his notable achievements was modelling photosynthetic processes with superconducting devices. Anton received his PhD in physics from University of Ljubljana in 2013. During his undergraduate studies he worked on exotic superconductivity and his work led to a discovery of a new type of matter called the Jahn-Teller metal.
Danny Wan is the program manager of quantum computing at imec where he coordinates the research and development efforts on qubits and cryo-CMOS. Danny joined imec in 2016 as an integration engineer in imec’s nano-interconnects program where he developed test vehicles for advanced metallization and alternative metals. Since then, he has led various projects including the development of a spintronic logic platform for imec’s beyond CMOS program and the industrial fabrication of superconducting qubits in the quantum computing program. Prior to joining imec, he worked at Intel Corporation as a process yield and integration engineer. Danny holds a PhD in physics from the University of California, Irvine for his research on single-walled carbon nanotube circuits and nanoelectronic devices.
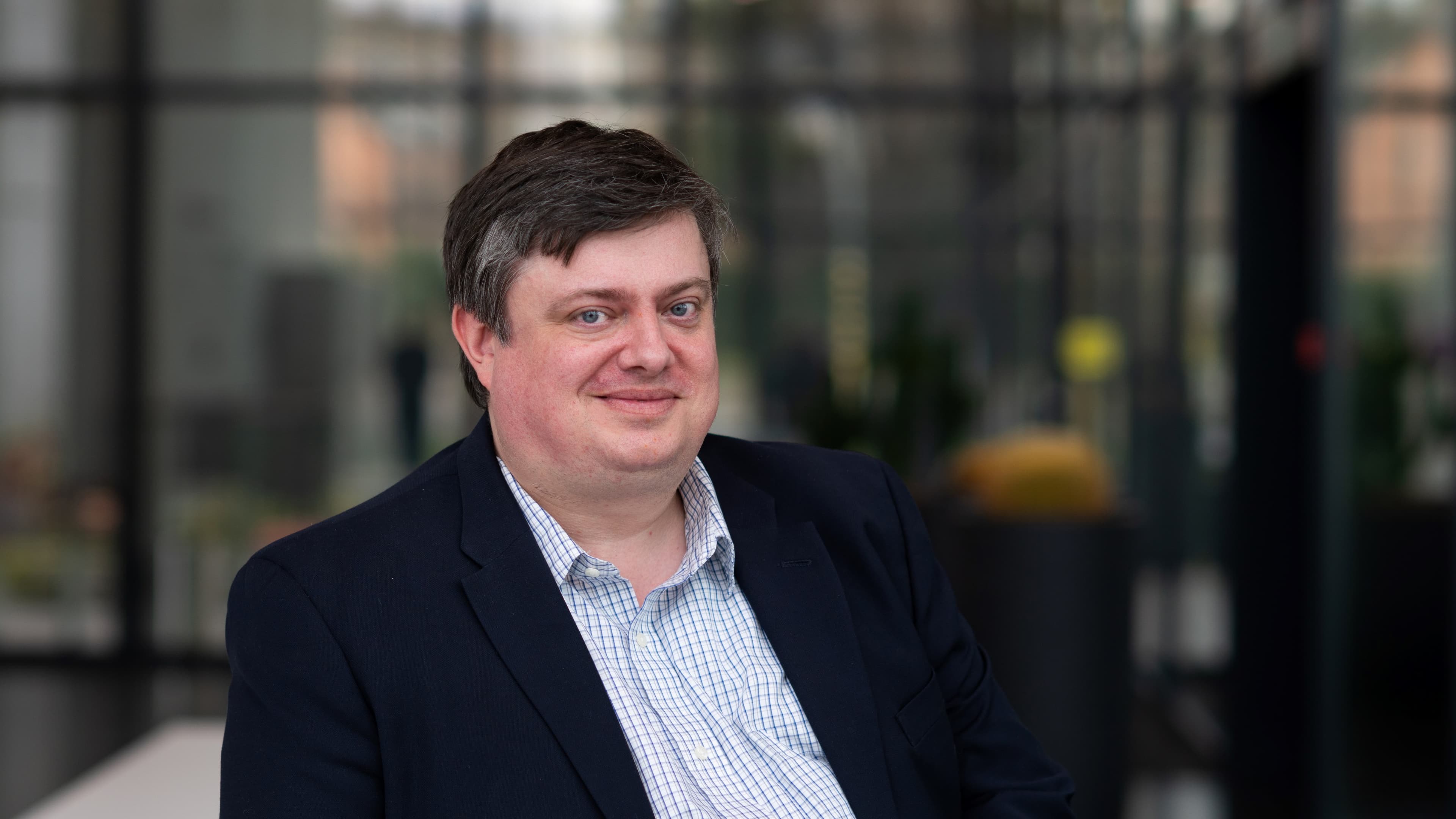
Kristiaan De Greve is a scientific director and program director of quantum computing at imec, as well as a professor of electrical engineering at KU Leuven. He joined imec near the end of 2019 from Harvard University, where he was a Fellow in the physics department and where he retains a visiting position. Kristiaan is an electrical engineering graduate of KU Leuven, and obtained his Ph.D. in Electrical Engineering from Stanford University in 2012 as a Stanford Graduate Fellow, with award-winning Ph.D. research under professor Yoshihisa Yamamoto on novel types of spin qubits, ultrafast means to manipulate such qubits, and pioneering experiments into the limits of light-matter qubit entanglement. At Harvard, he worked with Misha Lukin and Amir Yacoby to push the limits of magnetic quantum sensing and to develop the world’s smallest MRI system, and with Misha Lukin, Hongkun Park, and Philip Kim to develop two-dimensional semiconductors into a model platform for textbook quantum optics experiments in reduced dimensions. Besides his scientific interests, he holds additional physics, economics, and business degrees from Stanford and Harvard University.
Published on:
17 October 2022