Navigating the challenges of renewable energy prediction
The journey towards a sustainable future hinges on our ability to harness the power of renewable sources effectively. Solar energy, in particular, has emerged as a cornerstone of this transition. However, the unpredictable and variable nature of sunlight poses a significant challenge for accurately predicting energy yields. This uncertainty not only hampers innovation, but unnecessarily delays the sun’s use in global energy production.
While a solar cell's conversion efficiency is conventionally measured in a controlled environment, its real-world performance, influenced by diverse weather conditions, can significantly deviate. For stakeholders such as solar farm managers and energy providers, the paramount concern lies not in the cell's efficiency percentage, but in understanding its actual annual power generation in a specific location. With this (financial) yield uncertainty, the full potential of solar panels – and their integration into buildings, car roofs or agricultural applications – might remain unharnessed.
The pressing need for precision
Predictive models of energy yields currently exist, aiming to enhance accuracy and applicability across various photovoltaic (PV) technologies. But traditional 'grey box' models are often reliant on historical data and empirical insights, struggling to provide the precision required for optimizing solar installations.
In response to this pressing need for precision, imec’s Energy Systems team at EnergyVille, Belgium, presented their own model in 2017. Unlike conventional approaches, the model adopts a bottom-up approach – it delves into the intricate interplay of light, temperature, and electrical dynamics within solar panels, with a physics-based model of their interactions. This holistic approach is crucial, especially as the renewable energy landscape expands into uncharted territories of integrated photovoltaics, such as integration in infrastructure.
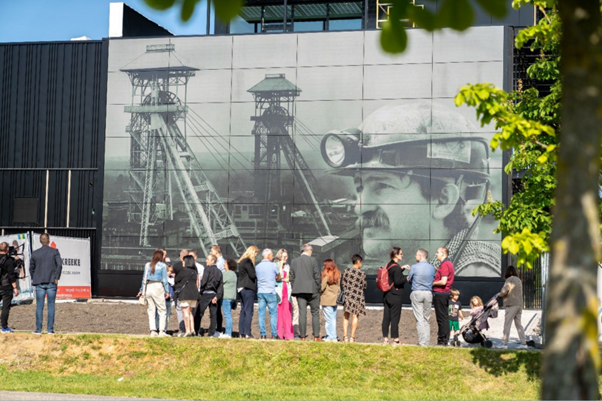
Facade of R&D production facility (Soltech), showcasing 42 inconspicuous building-integrated solar panels.
Understanding the Energy Yield Model: a physics-based simulation framework
The system model weaves together three interlinked components: an optical, a thermal, and an electrical model.
The optical model employs sophisticated 'ray tracing' techniques, simulating how solar modules respond optically. By also considering reflection or absorption across various wavelengths and angles, the model excels in capturing the nuances of sunlight interaction with different panel technologies. Secondly, the thermal model takes a leap beyond global module temperature estimates for solar panels, by factoring in local variations. This distinction is particularly significant for building-attached installations, where adhesion to e.g., concrete walls can be done seamlessly or by including space for airflow. Understanding these local ‘chimney’ effects is key to accurate temperature modelling and provides more nuanced predictions. Lastly, delving into the core of power generation, the electrical model considers various factors, such as the specific type of solar cell and the presence of (acoustic) absorbing material. It provides insights into the generated current and identifies potential areas of efficiency loss.
“The synergy of these three models, responding to environmental inputs like meticulous meteorological data and positioning, paints a comprehensive simulated 3D picture. Besides the straightforward impact of direct sunlight, factors like elevated temperatures and diminished wind currents can adversely impact solar panel yields. The model’s focus on local meteorological intricacies ensures a more accurate and dynamic representation of real-world conditions, enabling the model to predict the daily or yearly energy yield of solar cells under varying meteorological and irradiation conditions”, emphasizes Prof. Michaël Daenen, Principal Investigator at imo-imomec/EnergyVille.
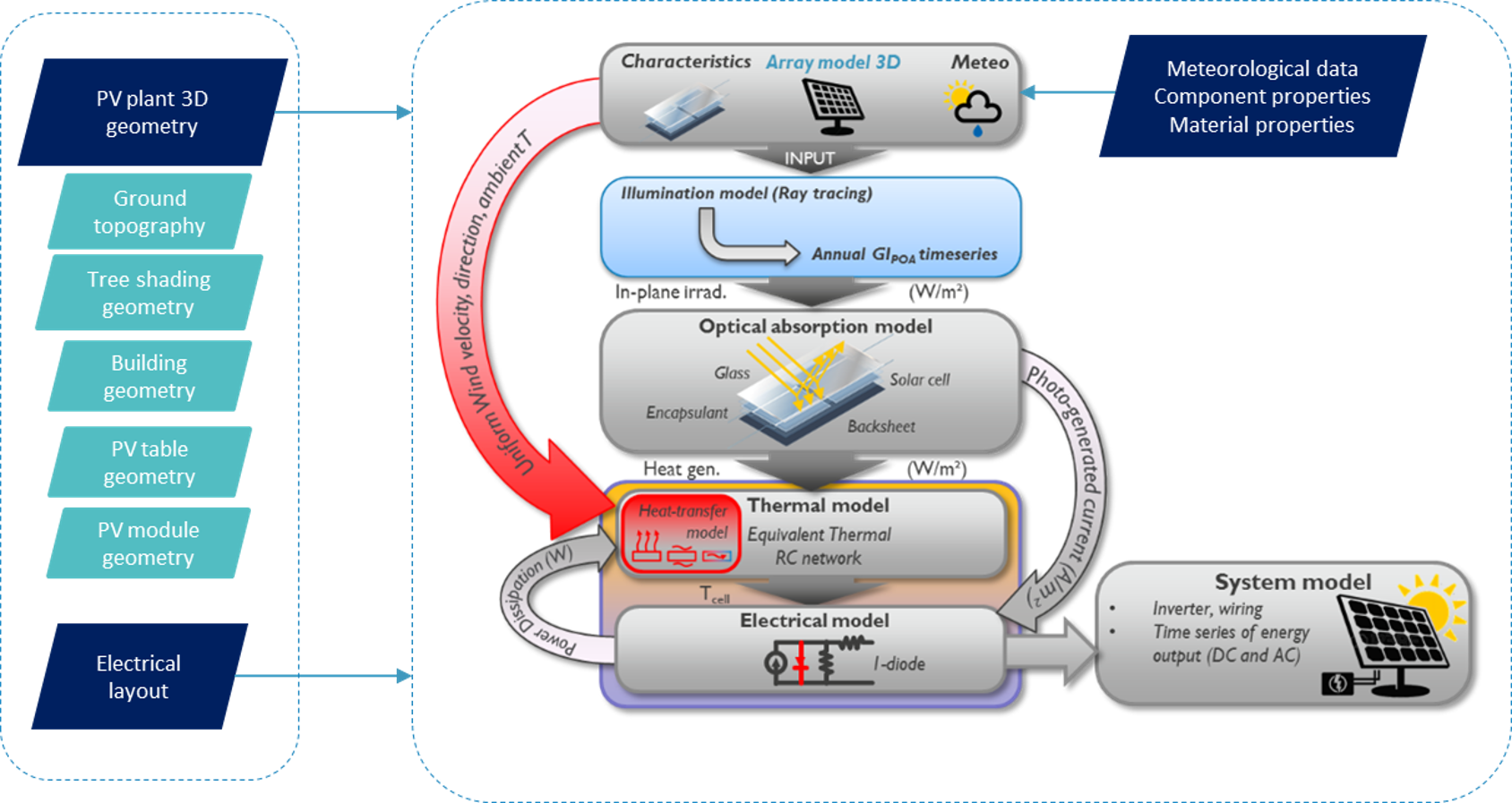
Flow of the physics-based energy yield simulation framework
From theory to real-world validation
Beyond theoretical simulations, the Energy Yield model has since served as a practical guide for optimizing PV integration – with experimental energy yields being compared to the simulated ones.
The Interreg Rolling Solar project, for example, focused on the integration of PV systems into public infrastructure, envisioning large-scale electricity generation without the need for additional land. Amongst others, a concrete noise barrier wall was built at EnergyVille, Belgium, in which existing and newly developed solar modules were incorporated, to showcase their feasibility and measure their energy yields over the course of several years.
Prof. Ivan Gordon, Department Director at imo-imomec/EnergyVille, explains the energy yield results:
“Our physics-based model gave remarkably accurate predictions, especially for crystalline silicon solar panels, within a 15-minute window. With over 95 percent of current PV installations being silicon-based, you can imagine the value of such accurate predictions. Challenges arose when we extended the predictions to thin-film technologies like CIGS modules. In response, we developed a separate electrical model for thin-film materials and included empirical corrections. With iterative refinement, we were able to significantly reduce the error (Root Mean Square Error (RMSE)) of our predictions to minor margins. This showcases the model's adaptability to different materials and technologies.”
In a second phase of the Rolling Solar project, modular bifacial solar panels were included in the wall, extending the model’s scope again. Whilst bifacial PV systems can generate up to 20% more electricity, compared to monofacial PV, their energy dynamics are significantly altered since photons can enter from both sides – highly dependent on the surroundings and varying throughout the day. These unique challenges were addressed with modifications in the optical model.
“The key strength of the Energy Yield Model lies in its flexible framework”, emphasized dr. Arnaud Morlier, team lead of the Energy Systems team at imec/EnergyVille. “This enables our model to evolve with real-world experimentation and progress in solar panel technologies and applications. It serves as a foundational framework that can be expanded to incorporate different materials and applications, providing insights into energy generation under different conditions.”
Beyond model optimization
A precise energy yield prediction of a specific application offers numerous advantages: a module engineer can assess the real-world impact of technical adjustments made to solar cells without the need for physical production. Additionally, a virtual presentation can aid in optimizing and testing scenarios for solar panel placement, considering factors like angle adjustments. From the design of PV-integrated solutions to estimating its energy yield in a specific location and optimizing investment strategies, the Energy Yield model can be a guiding beacon throughout the entire journey. Some examples:
Together with the software company PVcase, imec has transformed the predictive model into a commercially available simulation software, tailored for solar parks. The software is versatile, incorporating bifacial and other state-of-the-art technologies, allowing for easy design and accurate prediction of the energy yield in PV power plants.
The predictive model continues to play a crucial role in designing and optimizing curved solar panels for car roofs. Recognizing that current solar roofs offer only a limited increase in driving range, the SNRoof project integrated highly efficient solar cells into car roofs. Simultaneously, the HighLite project explored the development of cost-effective solar car roof modules, aiming to contribute to a competitive EU PV manufacturing industry. Currently, the Energy Systems team is evaluating the optimal placement of solar cells to facilitate the development of fully autonomous electric vehicles.
More recently, building on the success of Rolling Solar, the SolarEMR project was finalized. This 18-month endeavour focused on demonstrating cost-effective automated PV module production and electric interconnection of solar cells, for both building and infrastructure integration. Interestingly, the project also considered aspects beyond technical and financial optimization, such as regulatory frameworks and business viability, paving the way for large-scale PV projects. By collaborating with energy market providers, the simulation model was used to not only create more efficient solar cells, but also improve installation techniques, and micro-grid stability – a collaborative effort thus enabling quicker green solutions.
Take, for instance, the profitability of investing in solar-integrated sound barriers—a venture that hangs in the delicate balance of their placement. With roads winding and meandering, at times veering away from the sun's rays, the integration of PV technology into sound barriers may not always be a straightforward choice. Enter the Energy Yield model, offering insights for the seamless execution of such large-scale projects based on intricate road maps, solar cell properties, and local weather conditions and regulations.
A beacon for a sustainable tomorrow
Beyond design and optimization, an accurate virtual presentation of PV-integrated applications can also aid in its operation and maintenance. When an experimental energy yield is not reaching its simulated one, this can indicate e.g., damage to the solar panels or overgrowing grass which needs to be cut, offering a way to detect anomalies. This enables a centralized operating system, reducing the need to visit the solar-site – beneficial for remote or isolated solar farms.
Ongoing projects utilizing the prediction model involve the improvement of accurate weather forecasting (E-TREND) and ‘nowcasting’ (TRUST-PV) with the help of sky-imagers and artificial intelligence (AI).
The prospects for precise solar energy modelling hold immense promise, spanning from individual installations to entire energy networks. Finally, consider this: to guarantee its stability, it is crucial for supply and demand to always be balanced. A simulation model predicting energy yields with a 15-minute window of accuracy, can not only assist in grid management for large manufacturing facilities or urban areas, but can also enable countries to trade energy more efficiently on the international market.
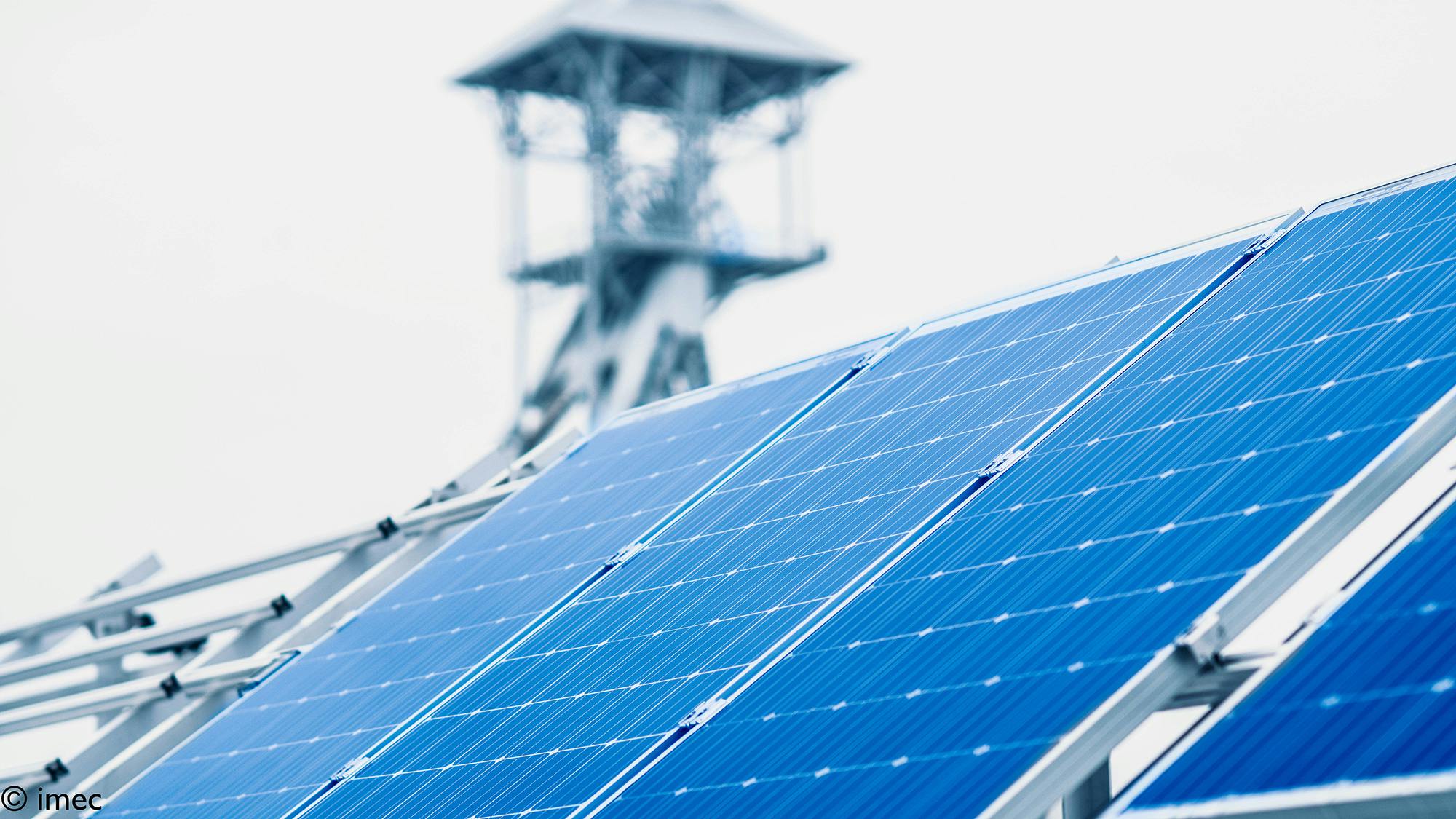
Bifacial Solar Cells at EnergyVille
The Rolling Solar and SolarEMR projects were collaborative efforts, carried out within the context of Interreg V-A Euregio MeuseRhine, with funding from the European Regional Development Fund and additional financial support through the Province of Limburg.
The HighLite 2020 project was coordinated by imec/EnergyVille and received funding from the European Union’s Horizon2020 Programme for research, technological development, and demonstration.
SNRoof in an imec.icon research project funded by imec and the Flemish Agency for Innovation & Entrepreneurship. The imec.icon SNRoof consortium consisted of industry partners AGP eGlass, IPTE Factory Automation, Arkema France Sa, and Michiels Group, as well as the research centers imo-imomec and imec/EnergyVille (TFPV, PVCM, Energy systems research groups).
This article also appeared on Science X - Tech Xplore news.
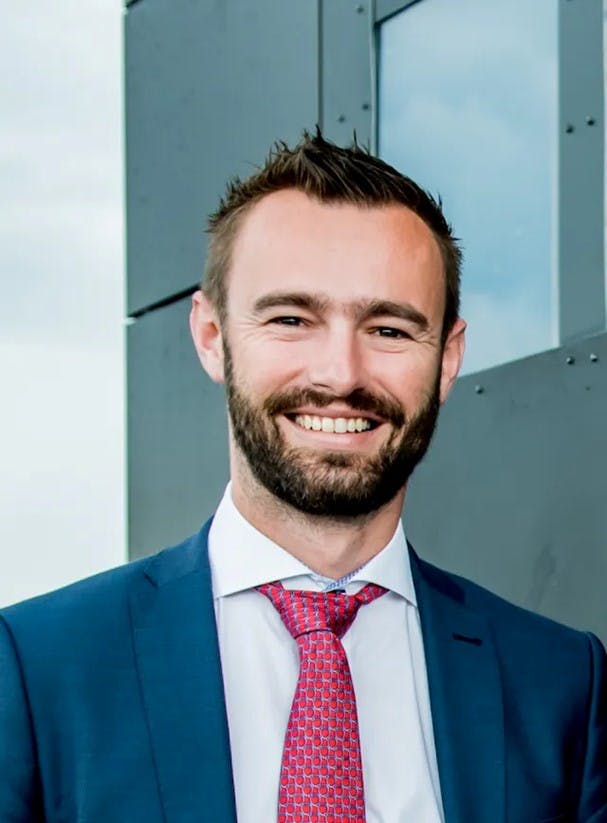
Prof. Michaël Daenen obtained his Ph.D. degree in 2008 in Material Physics, focusing on nanocrystalline diamond synthesis and characterisation, from Hasselt University, Belgium, where he currently holds the position of Professor of Engineering Technology. Michaël’s research activities are situated in the Energy Systems team and Wafer PV team at imo-imomec (an imec affiliated research institute) in EnergyVille, focusing on the reliability of PV modules and -systems. He is currently involved in several EU (Percistand, MC2.0), Interreg (Sunovate, SolarEMR) and Transitiefonds-funded (BREGILAB, MarineSpots) projects.
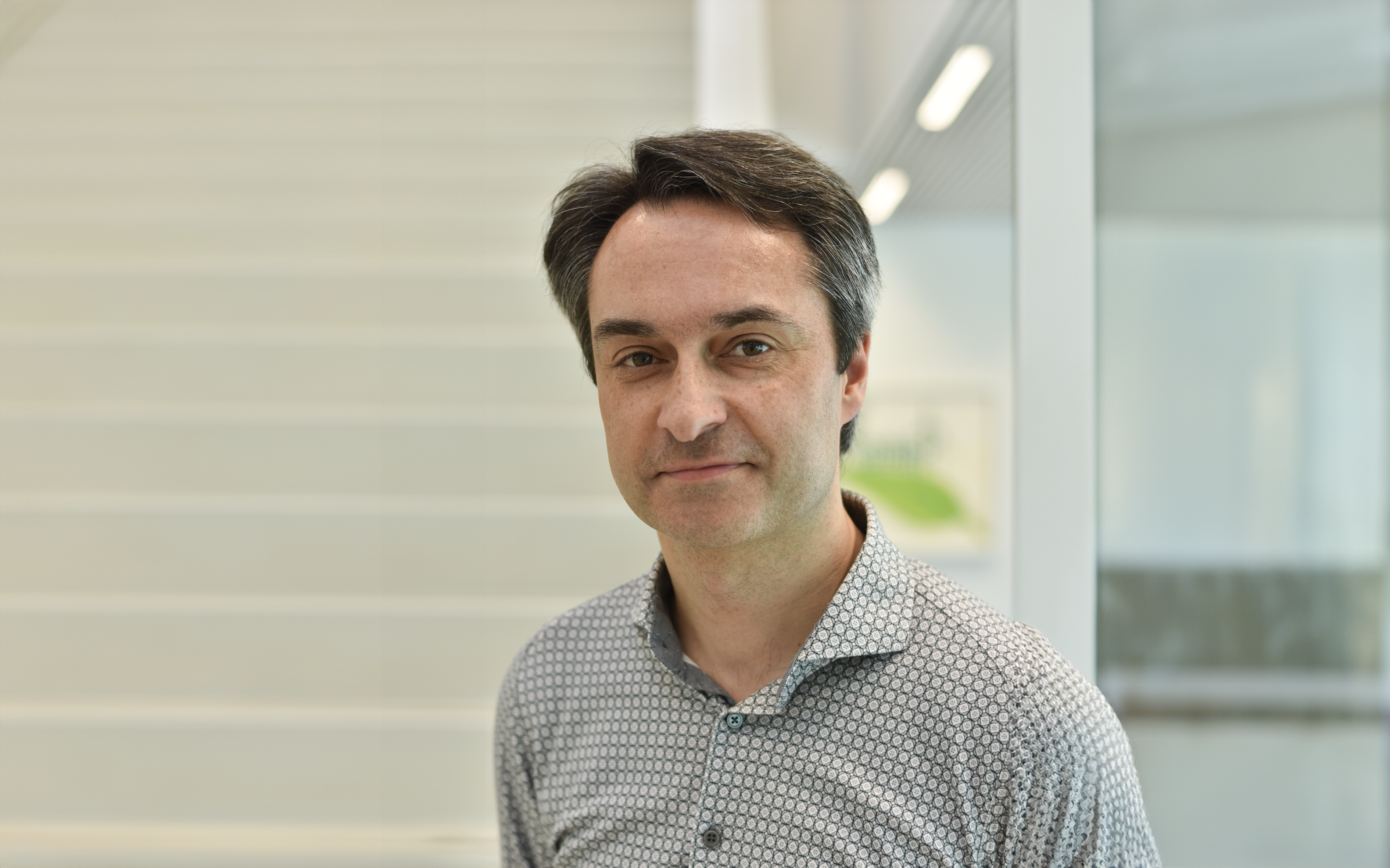
Prof. Ivan Gordon obtained his PhD in the field of novel magnetic materials for sensor applications from the KU Leuven, Belgium, in 2002. Transitioning to photovoltaics in 2003 at imec, he currently serves as the Director of the imec/imomec department in EnergyVille, and Vice-Director of the Institute of Material Research (imo) of Hasselt University, Belgium. Next to this, he is a part-time professor in Digital Photovoltaics at Delft University of Technology, the Netherlands.
Prof. Gordon is the Editor-in-Chief of the international scientific journal Solar Energy Materials and Solar Cells. Since 2016, he has coordinated the joint program on Photovoltaics of the European Energy Research Alliance (EERA) and a steering committee member of the European Technology and Innovation Platform Photovoltaics (ETIP-PV).
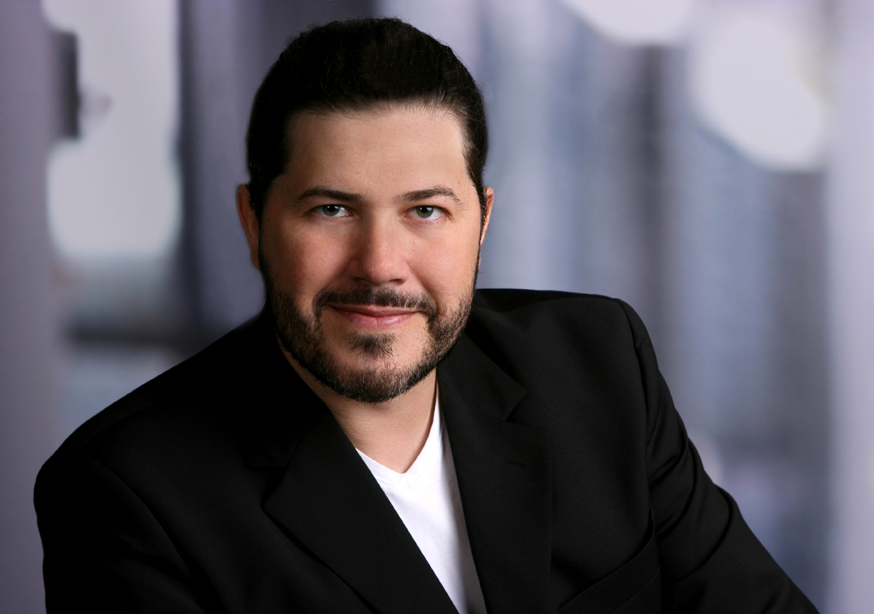
Dr. Arnaud Morlier obtained his Ph.D. degree from the University of Grenoble-Alpes in the field of flexible gas-barrier materials for optoelectronics in 2011. He began working in the field of photovoltaic modules reliability in 2011 at ISFH, Germany. Since 2022, he has been leading the Energy Systems team at imo-imomec in EnergyVille, Genk, Belgium.
More about these topics:
Published on:
20 March 2024