A new era of cancer treatment
Immunotherapy is considered to be the next big thing after the development of chemotherapy 40 years ago. Other than chemo and radiotherapy, immunotherapy holds the promise to achieve complete, long-lasting remissions and cures for all types of cancer.
One type of immunotherapy is cell-based immunotherapy, using the patient’s own immune (T-)cells, adapted in a way to better fight cancer. The latter is done by engineering the T cells with a specific T-cell receptor that specifically binds the cancer cells. Of these, the so-called CAR T cells, with a chimeric antigen receptor (CAR) on the cell surface, holds the most promise. Whereas CAR T cell therapy was first restricted to small and often experimental clinical trials in patients with blood cancer, it now resulted in the first FDA-approved therapies for two types of blood cancer.
The cost of these therapies is enormous: a few 100,000 dollar. This is related to the cumbersome workflows and regulatory requirements to proof high quality, reproducibility and effectiveness.
The cell therapy workflow
CAR T cell therapy is based on a patient’s own immune system. For this reason, the workflow starts with taking blood from the patient, typically in a hospital, selecting the white blood cells, and from this the T cells. Next, the isolated patient T cells are transported from the collection site, to a manufacturing facility. Here, the T cells are re-engineered to produce receptors on their surface that allows the cells to now recognize and destroy cancer cells. The code for the new receptor is inserted into the T cells through viral vectors or electroporation.
These re-engineered T cells are then cultured in bioreactors for expansion into numbers ordering in the hundreds of millions. Finally, the re-engineered cells are re-introduced in the patient, where they have the ability to further multiply and kill cancer cells.
Schematic of the CAR T cell therapy workflow
Next to the biological issues (side effects, efficacy for solid tumors, new tumor targets, ...), a key challenge is related to the manufacturing process. In patient-specific cell therapy, the complexity of the end product – living cells with a specific product profile – and the variability of the starting material (the patient’s own cells) make it challenging to ensure a cell therapy product that is comparable in quality and in terms of both safety and efficacy. Also, there is the complex logistics chain with blood being taken from a patient at a hospital or center, being cryopreserved, shipped to a facility where it is reprogrammed and manufactured in the lab, and then shipped back for infusion into the patient. And, there is the need for a short turn-around time due to the patient’s situation and evolution of his disease. And the therapy should be affordable by keeping cost of goods (CoGs) down and more importantly have a scalable sustainable manufacturing process.
How nanoelectronics can innovate the cell therapy workflow
The complexity, cycle time and cost of the CAR T cell therapy workflow is a major challenge to overcome in order to achieve clinical implementation of this revolutionary new cancer treatment. Chip technology can help to achieve this.
Over the last few decades, the semiconductor industry has grown exponentially, poised to increase value to the end-user while driving down costs by scaling. The result is the world’s highest standard in precision and high-volume production of nanoelectronics chip-based solutions.
Imec has used its semiconductor process expertise and infrastructure to make significant innovations in single-use silicon biochip and microfluidic technology, creating toolboxes of on-chip functions spanning cell sorting, single cell electroporation, integrated biosensors, and enzymatic assays key to addressing the challenges in CAR T therapy. These existing demonstrations on chip could enable to provide smarter solutions for discrete unit operations for complete CAR T workflow integration from T cell isolation from the patient all the way to reintroduction of therapeutically modified cells to the patient. Solving these challenges would enable more patients to access and benefit from the next most anticipated class of life changing therapies.
Workflow for CAR T cell therapy with indication (in blue circles) of potential nano-electronics impact (Figure inspired
by Regenerative Medicine Innovation Platform. www.smarthubvlaamsbrabant.be/projecten/regmed-platform)
Examples of relevant chip-based technology that imec develops are:
A bubble-based jet flow sorting technique
Imec developed an alternative sorting technique – a microfluidic FACS technique – based on the use of micro vapor bubbles generating a jet flow for high speed, but gentle, cell sorting in microfluidic channels. The technique combines high throughput, high precision, flexibility, assured biosafety and affordability. The sorting speed is 5,000 cells/sec per single microfluidic channel, with a > 90% cell sorting yield, > 99% purity and well preserved cell viability. Compared with other microfluidic cell sorting techniques, the bubble jet sorter is a generic cell sorting technique which is independent of any cell physical characteristics such as size or compressibility. This cell sorter technology has many advantages over the current cell therapy standard for selection, IMS: multi-marker support; more compact and automated; low disposable cost; no post-sorting step needed
A micro-electrode array for single-cell electroporation
Imec develops very large scale microelectrode arrays (MEA) for single-cell electroporation. These are silicon chips with thousands of small electrodes, covered with surface chemistry to make it compatible with cell cultures. When a small voltage is applied to the cell (via the electrode underneath), the cell membrane opens up and molecules in solution enter the cell. In this way, one is sure that every cell is transfected. The voltage used in these electrodes is so small that it has no negative effect on the cell. A second advantage (next to the low voltage) of the MEA-based single-cell electroporation is that there is a very precise control on the electroporation parameters for each individual cell, that can be verified one by one. This will most likely enhance the precision of the technique, resulting in more transfected cells, which are in good condition too. This will increase the yield and reproducibility, minimize the potential toxic effect and increase the ultimate efficacy of the cell therapy in the patient.
Ion sensors
After gene transfer, the CAR T cells have to be multiplied to millions of viable cells. This happens in bioreactors. It is key to carefully monitor and control what happens inside these bioreactors in order to achieve a cost-effective, robust and uniform commercial product. Measurement of parameters such as pH and dissolved O2 and CO2 provide information on the micro-environment of the cells. Imec develops multi-ion sensors for fluid monitoring, which can measure e.g. pH, Cl, Na, K, Ca, and NO3. It is a generic platform which can be tailored towards specific applications: by changing the selective membranes on the electrodes, the sensor can be adopted to detect other ions. The sensors outperform current systems in terms of performance and are easy to mass-produce, have a wireless connection, are energy optimized and extremely miniaturized.
A miniaturized lens-free microscope
Also in the bioreactor, a visual inspection of the cells is indispensable to get direct feedback on the viability and overall condition of the cells. Normally this is performed by a process operator by taking a sample out of the bioreactor and inspecting it with a microscope. Imec has been developing a lens-free imaging cytometer technology that could be integrated on top of microfluidic channels, enabling in-flow cell imaging in microfluidic channels, or into bioreactor walls. The lens-free holographic-imaging based system uses a LED or LASER light source and a CMOS imager to capture the light that is diffracted off small objects. The captured diffraction pattern (called a hologram) resembles the ripple effect pattern caused by an object landing on a water surface. Custom software algorithms are required to reconstruct the hologram into an in-focus image that resembles the object that is being imaged. It is a compact solution, which enables a large field of view and very good resolution, at an affordable price. A machine-learning based image analysis and classification pipeline is also being developed to evaluate the images and differentiate between specific cell types using powerful classification algorithms.
Waveguide-based biosensor assays
Again in the bioreactor step of the workflow, it is important to analyze by-products of cell metabolism – proteins and enzymes. Current technologies e.g. based on immunoassays (mostly ELISA), mass spectrometry, chromatography or spectroscopy are not yet available in on-line setups, require large efforts in sample preparation and often lack specificity in complex culture media with a high protein background. As an alternative, imec develops photonics-based immunoassays. Photonics is a well-known technology used in e.g. glass fibers to transport data in a more efficient way. But photonic waveguides and other components can also be used for life science applications. To enable this, imec – together with partners in the European PIX4life project – has set up a silicon nitride – for visible light – photonics pilot line. A full library of photonic components is available for life science companies to build the next generation of photonic biochips. With this photonics platform – and more specifically with the use of photonic waveguides – imec has built a fluorescent immunoassay and an enzymatic colorimetric assay which could be used for online monitoring of relevant cytokines in bioreactors for CAR T cell expansion.
Save time and lives with a chip-centered workflow
With a microfluidic-based and chip-centered approach, it is expected to have a much shorter turnaround time (from T cell selection to infusion), for several reasons: (1) all process steps (selection, electroporation) are performed very accurately resulting in a higher yield of CAR T cells from the same amount of blood; moreover, this would eliminate the differences in efficacy and toxicity as seen today in clinical trials; (2) by using microfluidics, processes occur much faster (e.g. cellular reprogramming with microfluidics occurs 50 times faster than traditional reprogramming); (3) chip processing enables a high level of parallelization, speeding up the process enormously.
It is the unique partnership between chip technology and pharma that can lead to innovative results, also in the field of cell therapy.
Want to know more?
- For more details on the chip-based technology that imec develops for cell therapy, download the whitepaper on this topic.
- Read the article on the PIX4life project to learn more about the silicon nitride photonics platform for life science applications.
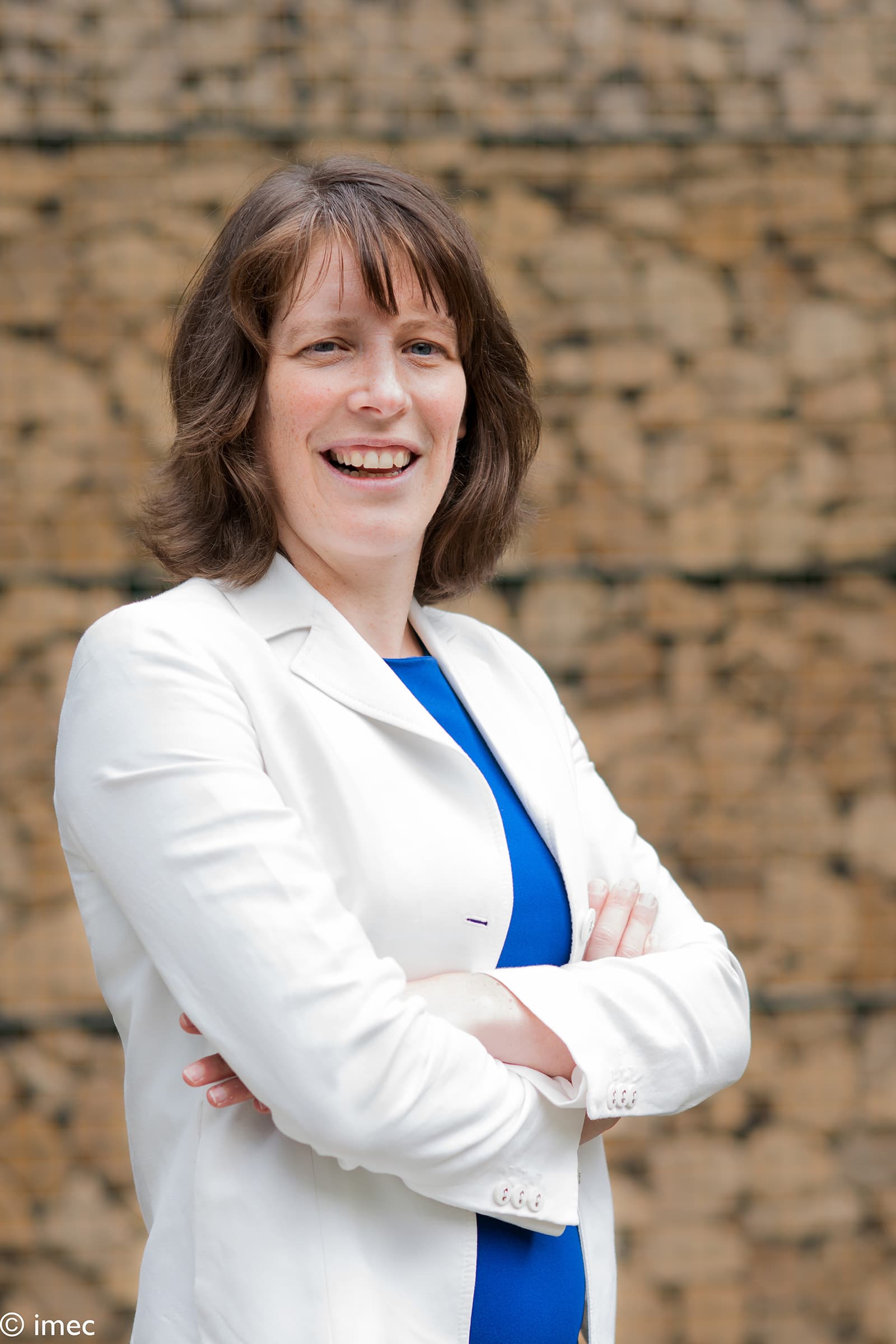
Liesbet Lagae is co-founder and Program Director of the Life Science Technologies in imec. In this role, she oversees the emerging R&D, the public funded activities and early business creation. She holds a PhD degree from the KU Leuven, Belgium for her work on Magnetic Random Access Memories obtained under an IWT grant. As a young group leader, she has initiated the field of molecular and cellular biochips leveraging silicon technologies at imec, Belgium. The life science program has grown from emerging activities to a mature business line that provides smart silicon chip solutions to the life science industry. Applications include medical diagnostics, point-of-care solutions, DNA sequencing, cytometry, bioreactors, neuroprobes, implants. She holds a prestigious ERC consolidator grant for developing a platform on single cell analysis and sorting. She has (co-) authored 125 peer-reviewed papers in international journals and holds 15 patents in the field. She is also part-time professor in nanobiotechnology at KU Leuven/Physics department.
Published on:
1 February 2018